On February 1, 2013, Hiroshima University established two new programs: the “Distinguished Professors” (DP) program and the “Distinguished Researchers” (DR) program. Individuals who are part of these programs are recognized as senior and junior faculty members respectively, who are engaged in extraordinarily distinguished research activities.
Interview of Distinguished Professor Kenta Shigaki
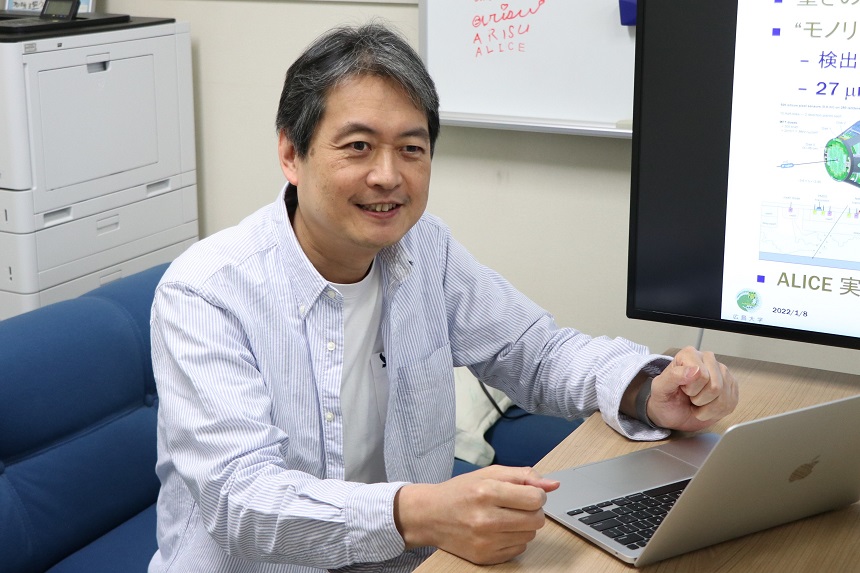
What Was Happening in the Fireball Created in the Instant of the Big Bang?
We're Experimenting to Discover Just How the Universe Was Formed
Turning Back Time to the Birth of the Universe
I specialize in high-energy nuclear physics. I am researching the kinds of substances that filled the universe soon after the Big Bang, and we do this by recreating in the lab the "fireball" that emerged in the Big Bang's immediate aftermath. Ultimately, our goal is to discover through these experiments just how the universe was formed.
When the Big Bang occurred some 13.8 billion years ago, all the energy in the whole universe was condensed into a single, superhot point—a fireball. The universe began to, and continues to, expand and cool.
My research involves smashing high-energy nuclei together in a particle accelerator to recreate the high temperatures of the fireball. In taking something cool and making it superhot, our experiments reverse the process of the maturing universe—turning time back to the universe's beginning. We know that the higher the energy of the collision, the closer we get to the state of things at the moment of the Big Bang. We are hampered by the limits of current technology; the closest we can get is somewhere between one hundred-thousandth and one millionth of a second after the Big Bang. It is believed that quarks and gluons—the smallest building blocks of substance—still moved about freely at that stage of the fireball.
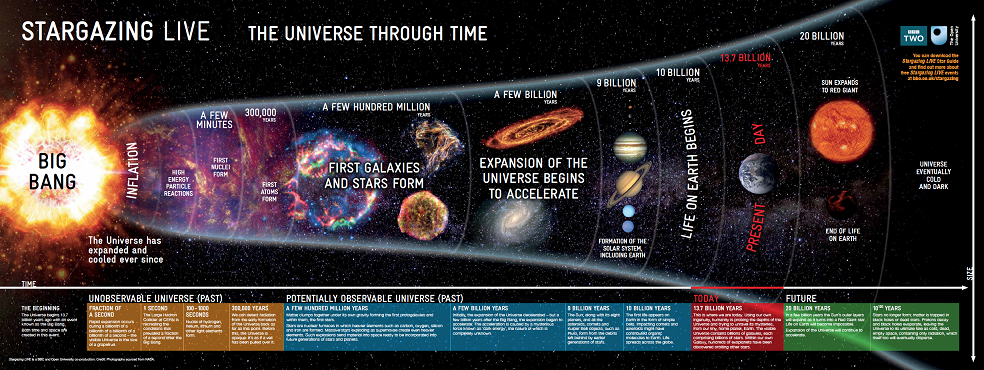
The history of the universe. ©The Open University, BBC.
Successfully Extracting Elementary Particles Using an Accelerator
The protons and neutrons that make up a nucleus are themselves made by bonded groups of elementary particles called quarks and gluons. Normally it is not possible to isolate these, but it is said that protons and neutrons can be melted and their inner particles set free at around two trillion degrees—roughly 100,000 times hotter than the center of the sun. Researchers everywhere have been trying to study this since the 1970s, but the sheer difficulty of generating such temperatures has hindered progress. It wasn't until the 1990s, around the time I began post-graduate study, that research into extracting the particles began to make headway. I leaped at the chance to study this largely undiscovered field, and have been at it for some three decades since.
The biggest outcome of my research so far came from a joint international project at the Brookhaven National Laboratory in the US in the 2000s. Using an accelerator called Relativistic Heavy Ion Collider (RHIC), we finally managed to see quarks and gluons in their separated state. Until then, it was believed that quarks and gluons behaved like gas, but we observed that they were more like a fluid. This was an electrifying discovery, so unexpected that one theoretical researcher likened it to Columbus setting sail for India and accidentally discovering America. That milestone did not mark the end of our research, but rather opened the field up to new discoveries as we probed ever deeper.
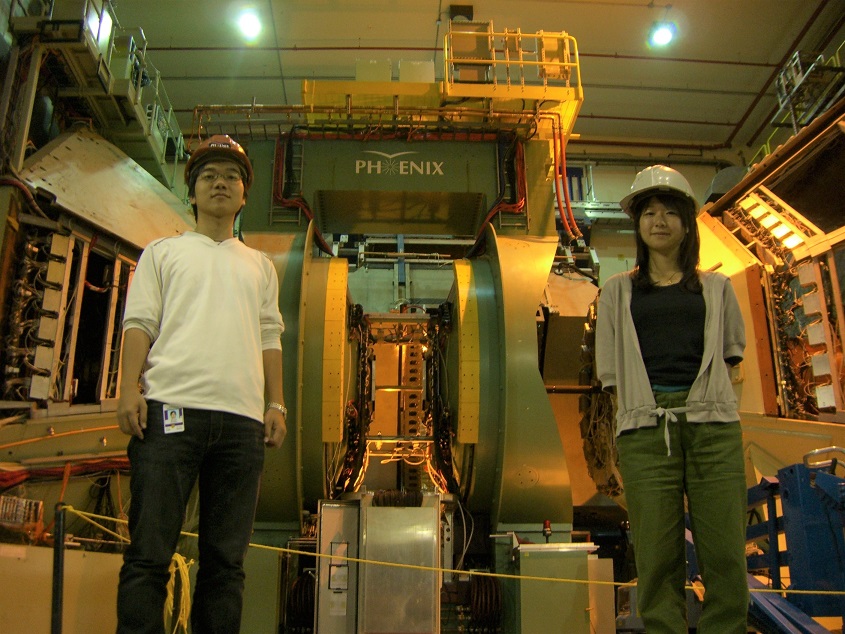
Hiroshima University post-grad students take part in the Phenix project using the world's first heavy ion collider RHIC at the Brookhaven National Laboratory in New York in the 2000s.
Next Quest: Discovering How Substances Got Their Mass
One of the greatest mysteries I'm trying to solve these days is the origin of the mass of substances. We know that when three quarks bond you get a proton, but the theory doesn't add up: the mass of an individual quark times three only amounts to around one percent of a proton's mass, which means that somehow, when quarks bond to form a proton, they become 100 times their mass. Yoichiro Nambu came up with this hypothesis way back in 1961 and we still haven't been able to achieve a clear answer in the lab. My hope is to use particle accelerator experiments to identify where the various substances in our vicinity get their mass—their "weight," if you will.
To do that, we need to measure the mass of the particles inside the fireball generated in the instant when nuclei collide. Theoretically, they should have become lighter, but it's exceedingly difficult to weigh them because particles that break free from their nuclei in that collision immediately begin to cool and revert to their original mass.
Researchers all around the world are working on this problem, many of whom are focusing on electrons. Electrons are comparatively easy to identify among the thousands and thousands of particles sprayed when nuclei are smashed together at high energy. However, when colliding nuclei together, huge volumes of electrons exist other than those generated by the decay of the particles we hope to observe, meaning there is a lot of extraneous noise to be contended with.
That's why I decided to target muons. Although they are harder to pick out at low momentum than electrons, there is less noise, meaning it is much more likely to achieve a clearer observation. And with our upcoming opportunity to focus on muons using the large hadron collider (LHC) at CERN in Switzerland, which enables higher-energy collisions than the RHIC, we are drawing closer than ever to unlocking the mystery of the change in particle mass. Work has just been completed on the development of a detection device, and we are scheduled to begin experiments with the LHC in the spring of 2022.
Meanwhile, in addition to studying the origin of mass, we are researching space's strongest magnetic field, which is also generated alongside the fireball. Making sense of the phenomena that occur in a world far beyond what most of us can imagine enables us to understand the building blocks of our universe and the mechanisms of its birth.
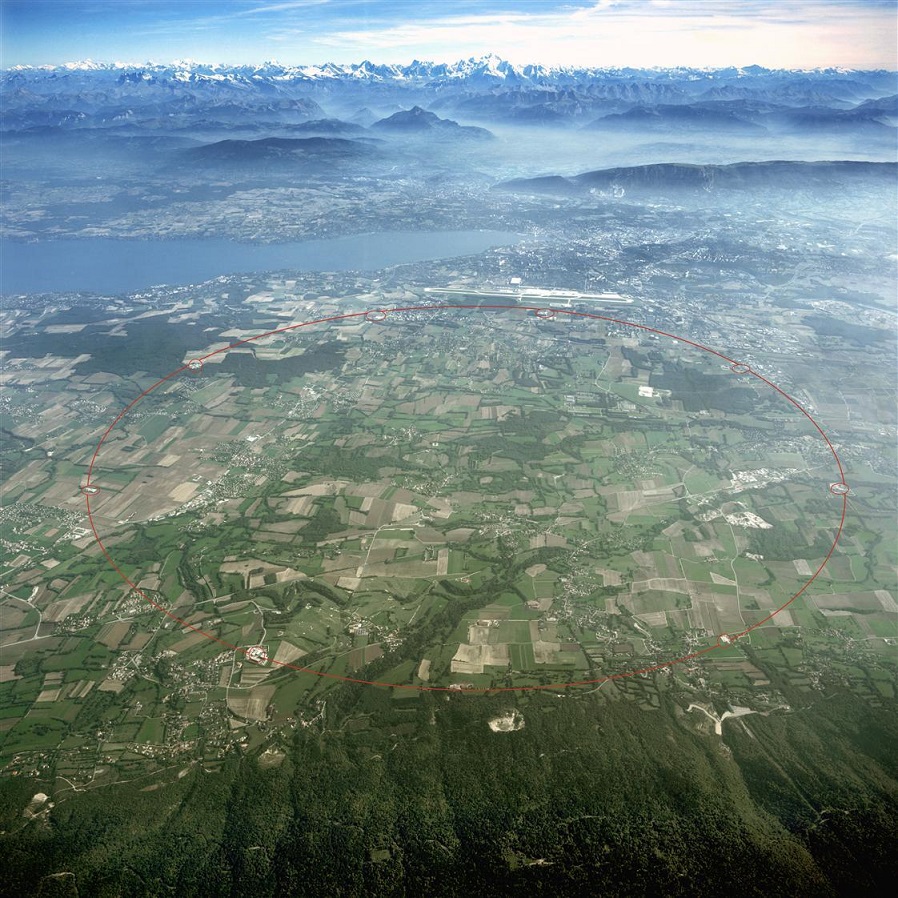
The LHC, the world's highest-energy particle accelerator, is situated in a tunnel under the Switzerland-France border. ©CERN